A recent study by Beatrice Hahn and colleagues, published in Nature Communications, has solved a major puzzle about the origin of one of the parasites that causes malaria in people worldwide: Plasmodium vivax.
Malaria is one of the world’s deadliest diseases, killing perhaps a million people each year. Most of the people who die from malaria are in Africa, and most of them children. In areas where malaria is common, adults often have a degree of resistance to the disease, but still get sick enough now and then to miss many days of work, suffering from agonizing aches, fevers and chills. Even though most victims are children, many adults die as well, especially when their immune systems are weakened by other infections. Malaria thus has huge economic costs and has been cited as one of the main drags on economic growth for many tropical nations.
Malaria has had a huge impact on human history and evolution. Malaria is one of the major reasons that Africa resisted European colonialism for so long. Europeans visiting Africa died in droves until the discovery that a drug extracted from the bark of the South American cinchona tree, quinine, protects against malaria. Quinine is fairly awful stuff: when I took it to fight a particularly bad malaria infection, it caused vomiting and a painfully loud ringing of the ears. From the mid-20th Century on, more effective anti-malarial drugs have been produced, which have helped many millions of people survive this terrible disease. Visiting and working in the tropics for business, tourism or, say, field primatology, would be a lot more dangerous without these drugs.
Before the discovery of such drugs, and for the many millions of people in poor countries who still don’t have adequate access to them, malaria has served as a powerful source of selection pressure on human populations. Because malaria is so deadly, human populations with long exposure to the disease have evolved a number of different defenses.
Malaria was originally thought to be caused by the “bad air” (Italian: “mala aria“) of swamps and marshlands. It is now known to be a group of similar disease caused by several different species of single-celled protozoans from the genus Plasmodium. Plasmodium falciparum is the most deadly, and the most common in Africa. Plasmodium vivax is more common in Asia. Because these parasites are different species, and only distantly related, tricks that work to defend against one species of parasite may not work agains the other.
The most famous anti-malarial adaptation is sickle-cell disease, which is caused by a single small change to a gene for hemoglobin, the protein that carries oxygen in red blood cells. This change changes the shape of the hemoglobin molecule.
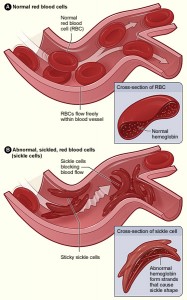
Red blood cells are packed full of hemoglobin, and if cells have only the abnormal hemoglobin, they become abnormally curved (shaped like a sickle or a crescent moon). People with one copy of the gene for sickle-cell disease have higher resistance to Plasmodium falciparum.
People with two copies of the gene, though, have sickle-shaped that cells get stuck going through narrow capillaries, causing all sorts of problems, which shortened life expectancy before the development of modern medical treatments.
Falciparum malaria is such a dangerous disease that having improved resistance more than offsets the risks of having children whose lives are shortened by inheriting two copies of the gene.
Nonetheless, sickle-cell trait seems a rather clumsy solution to the problem. Kind of like using hand grenades to protect yourself from tigers. The grenades can stop tigers fine, if you can throw them far enough. But if you don’t throw them far enough, they blow up too close and kill you instead. Perhaps sickle-cell is an emergency stop-gap measure that evolved too recently for all the kinks to be worked out yet.
A seemingly better solution to this sort of problem is the Duffy-negative trait, which provides resistance to a different species (Plasmodium vivax), with little apparent cost to people who have the trait.
To understand the tricks that have evolved to defend against different species of Plasmodia, it is useful to know something about the life cycles of these parasites. These life cycles are complex and involves different stages of sexual and asexual reproduction in various organs of different hosts, including the human liver, human red blood cells, mosquito guts, and mosquito salivary glands.
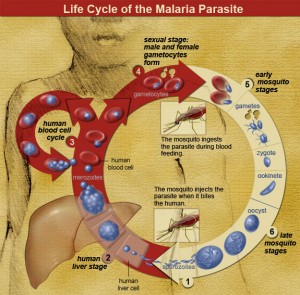
The malaria life cycle is a complicated solution to problems of replication, sex, and dispersal of Plasmodium genes. In most animals that we are familiar with, such as ourselves, individual organisms do the major work of replication, sex and dispersal. As individual organisms, we accomplish these goals by mating, raising kids, and sending them off to college, for example.
Malaria parasites have found ways to outsource most of this work to other organisms. To replicate, they turn human hosts into giant malaria factories, turning red blood cells into production centers that burst, releasing newly produced parasites into the blood stream, infecting new red blood cells, in an exponentially increasing production system that destroys millions of the host’s red blood cells. This is the part of malaria where the human host feels weak and miserable and suffers from alternating fevers and chills.
Most of this replication phase is asexual: making millions of copies of the same thing. This works fine within a single host, but if you want your babies to survive in the cruel and variable outside world, you need to boost their chances by introducing variation into their genes through sex. So towards the end of the cycle, the parasites start making male and female versions, the gametocytes. When mosquitoes suck the blood of an infected host, they suck up these gametocytes, which can then have sex with gametocytes picked up from other hosts. The offspring of the gametocytes then infest the mosquitoes gut, eventually sending sporocytes to the salivary glands, so they get injected into the next person the mosquito bites, starting the cycle over again. The mosquito thus serves as both a malaria parasite dating service and dispersal system. Kind of like college.
Anyway, a key part of the malaria parasites life cycle is getting into the host’s red blood cells. To do that, they use specific proteins on the surface of the blood cell, which serve to transport certain chemical signals across the cell membrane. Plasmodium vivax parasites use one specific kind of protein, the Duffy antigen receptor, to force their way into human red blood cells. In much of Africa, the indigenous people don’t have this receptor. There is thus no way for P. vivax to infect them. People who don’t have the Duffy antigen receptor may suffer some costs, such as increased susceptibility to asthma, but these costs don’t seem to be anywhere near as high as those imposed by sickle-cell trait. And of course they are much less than the costs of dying from malaria.
The African distribution of the Duffy-negative phenotype has been a puzzle, though, because P. vivax is very rare in Africa. The conventional wisdom has been that P. vivax evolved in Asia. How could a parasite that evolved in Asia, and is rare in Africa, select for parasite resistance in Africans? This would be as puzzling as if people in Africa were all born with some sort of inherent immunity to tiger attacks, even though there are no tigers in Africa.
Beatrice Hahn and her team have solved the problem by collecting poop from thousands of apes across Africa. I played a very small part in this study, by overseeing poop collection for a while at Gombe.
Humans are apes, and many diseases that infect humans can also infect other apes, or (like in the case of HIV) originally came from other apes. Malaria is no exception. On a molecular scale, we are so similar to chimpanzees and gorillas that the tricks pathogens use for getting into human cells often work for these cells in other apes as well.
Beatrice Hahn’s team has been collecting fecal samples from apes all across Africa as part of a study looking into the origins of HIV-1, the cause of the global AIDS pandemic. It turns out that the same samples, and same molecular methods, that are so useful for studying HIV, are equally useful for studying all sorts of other things that live inside humans and other apes, including other viruses and gut microbes.
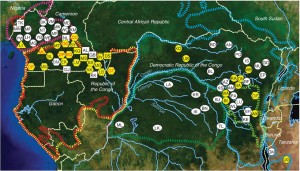
It turns out that when apes are infected with malaria, they shed some of the malaria DNA out with their poop. Take some poop, put it in a jar of RNAlater, and you can recover all sorts of fascinating genes, including malaria genes.
(When Jimmy Fallon and Justin Timberlake visited Gombe some years ago, they seemed amused by all of our poop collection and made up a song about it: “Poop in a Jar.” Disappointingly, though, they don’t seem to have recorded this one yet.)
It turns out that gorillas and chimpanzees across Africa, but not bonobos, have malaria parasites that genetically are very close to Plasmodium vivax. Compared to the gorilla and chimpanzee parasites, the human vivax is much less genetically diverse. All the human P. vivax belongs to a single branch of the much bushier tree of African ape P. vivax.
It thus looks very clear that P. vivax evolved in Africa, not in Asia, from a plasmodium population that infects other African apes. People must have carried P. vivax with them when dispersing from Africa some 60,000 years ago. More recently, human populations in Africa evolved the Duffy-negative phenotype that proved so effective that P. vivax became extinct in Africa.
This study underscores the importance of evolution in human lives. Evolution happens fast, and human populations continue to evolve in response to our environments. The parasites that infect us evolve even faster. Evolution leaves traces in the genomes of every living organism that can be used to solve innumerable fascinating puzzles. This study highlights the power of molecular methods to answer important questions, especially when combined with field studies.
Interesting study. If it weren’t for the association with miralaa endemicity apparently attested in Africa and Europe, the pure gene evolution would point to a migration out of America (ancestral state) through East Asia to Europe and Africa (derived state). India is a bridge between the east and the West and a “swing” continent. Africa still preserves the original state in some pockets such as Madagascar, Horn of Africa and northwest Africa but overall it has shifted away from the original condition. But even with the miralaa association in Africa and Europe this scenario would work.The map of Hbs distribution reminds me of Y-DNA YAP+ distribution, with Africa being heavy on YAP+ but with pockets of YAP-, Europe intermediary and Asia showing a strange small pocket of YAP+. India has Hbs, Tibetans and Andaman islanders have high frequencies of YAP+. America is devoid of YAP+ completely. There’s a parallelism here, I think.